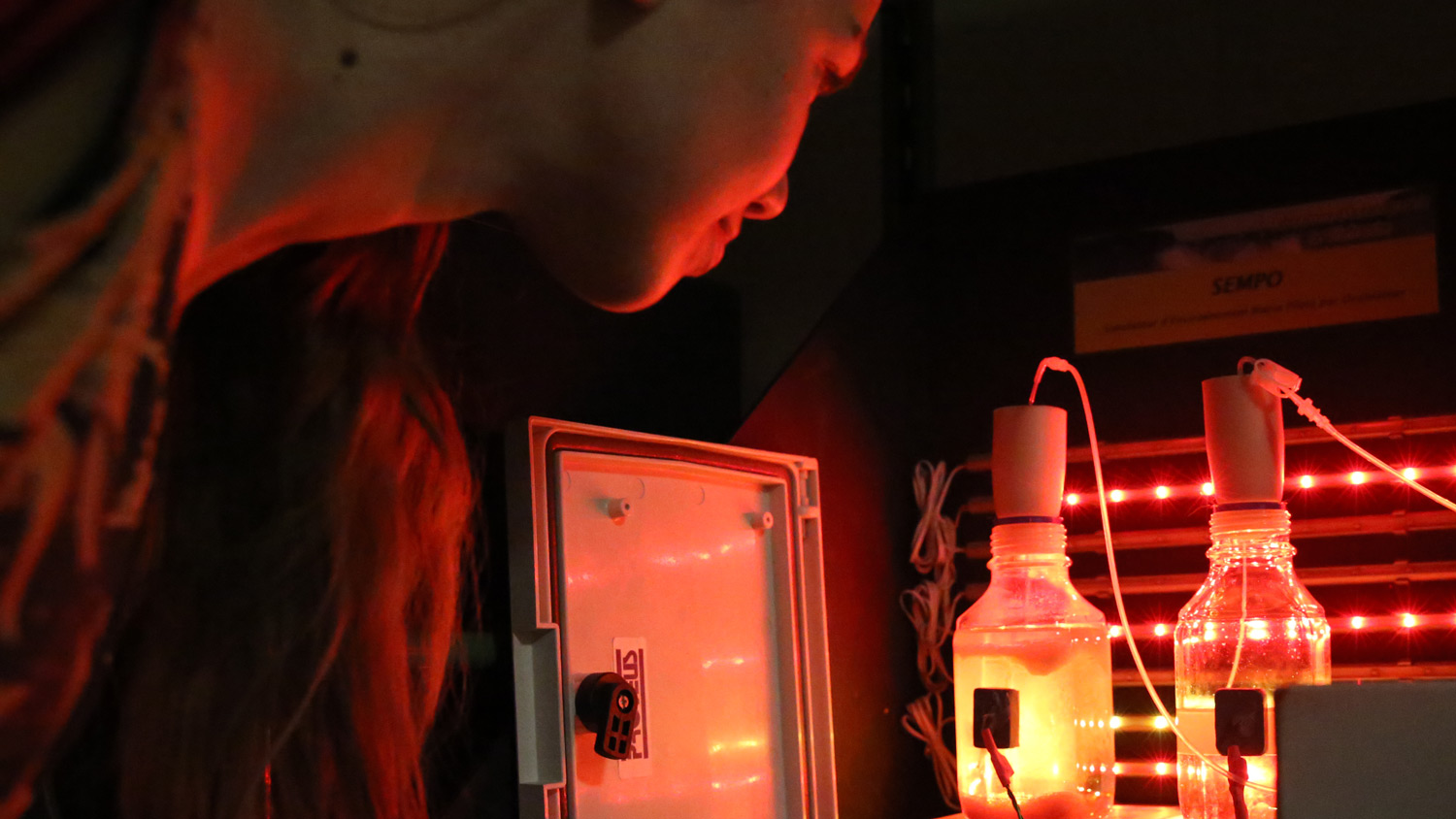
Growing up, Olivier Bernard wanted to become a forest ranger in order to observe trees in the great laboratory of life. But finding their growth too slow for his taste, the budding scientist eventually turned to the ocean and the fast-paced dance of microscopic algae instead. “In terrestrial ecosystems, living organisms orchestrate their own symphony,” Olivier explains. “Each part knows its role, allowing the whole to thrive. This built-in consistency does not prevail in aquatic ecosystems, where only an external hand can mastermind novel and complex collaborations. This is both boundless and fascinating!” What Olivier was yet to find out was that his passion turned expertise would prove critical in facing yet another accelerating pace: that of climate change.
Now leading an interdisciplinary research team spanning four research institutes (CNRS, Inrae, Inria, and Sorbonne Université), Olivier isn’t done demanding quick answers for both current and future generations. His team’s mission could be summarized in two action verbs: to model and to optimize. First, taking into account the impact of climate change, they will use mathematical models to predict the microscopic biodiversity of ocean ecosystems by the end of the century. Technology and biology will then team up to offer optimal strategies to mitigate and even counteract our harmful impact on the environment.
Understanding phytoplankton plasticity to predict the ocean's future
To change the world, you first need to understand what it might become. According to the team, mathematical models have everything they need to predict the course of life underwater. Working from the five scenarios provided by the IPCC, they are conducting innovative experiments at the Laboratoire d'Océanographie de Villefranche-sur-Mer (LOV) to evaluate the plasticity of microorganisms and their ability to adapt to climate change.
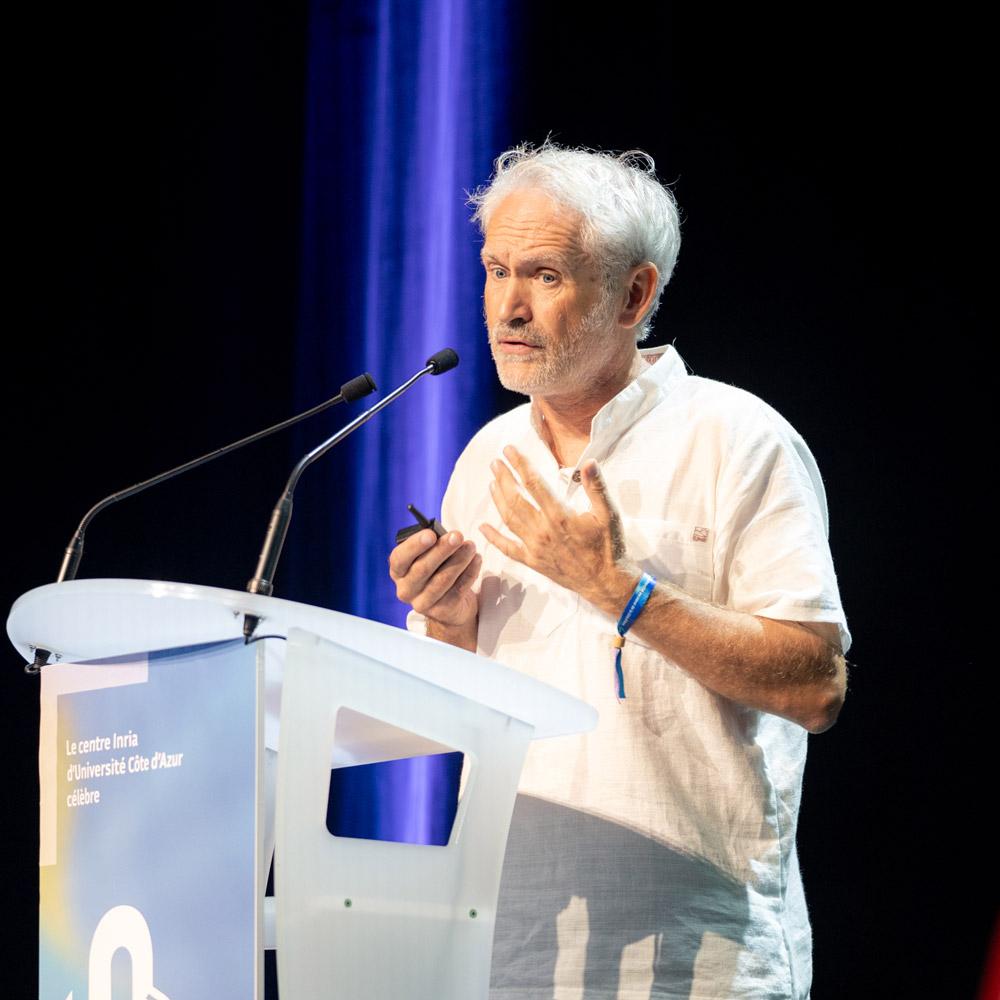
Verbatim
Why are we so interested in bacteria and phytoplankton? Primarily because of their ability to quickly adapt to change. By placing them in bioreactors – environments that we can control using technology – we can subject them to pressures similar to those they will face in the ocean as a result of climate change, and observe their adaptation over the span of a few years. In other words, we predict their future using the most efficient crystal ball of our times: computational modeling.
Research Director, Centre Inria d’Université Côte d’Azur
There is yet another reason for the team's keen interest in phytoplankton: their role as first link in the food chain. Tiny but mighty, these microalgae introduce the first surge of energy and matter into the vast network of food chains within marine ecosystems. By modeling the impact of CO2 emissions on phytoplankton biodiversity, the team can thereby predict whether life will thrive abundantly within a given aquatic ecosystem or not. Either the pace is too high and phytoplankton can't cope, species run out of food and monospecific stands prevail in the end. Or microorganisms end up adapting better than expected, generating more biodiversity. “Both scenarios will arise depending on the region of the globe,” Olivier explains. “Our role is to map out the ocean’s future based on microorganism plasticity, although we already fear more biodiversity loss than gain along the way.”
Controling ecosystems with AI
The second mission of Biocore is best explained by environmental engineer Francesca Casagli on the TEDx Cannes stage. Computational modeling allows her to see the full complexity of the invisible, as well as to simulate all possible microbial and algal collaborations and their consequences. Oceanographers Antoine Sciandra and Lionel Guidi from the Oceanography Laboratory of Villefranche-sur-Mer conduct experiments and measurements at sea to validate these models and enhance their predictive power. To handle the massive amount of data collected, artificial neural networks take over. Without them, key information hidden in this data would slip through the limitations of the human brain.
The team is putting all branches of science on their side because time is of the essence. Phosphorus and nitrogen are rare and therefore precious resources. Nitrogen, an essential ingredient for building proteins in the human body, is equally crucial for agriculture. But producing 1 kg of nitrogen fertilizer from atmospheric nitrogen currently requires 1 kg of oil. As for phosphorus, its terrestrial reserves are shrinking all the faster as demand for car batteries has reached an all-time high.
The challenge is therefore environmental, economic, and monumental. Bioreactors are highly sophisticated factories, filled with self-dividing cells that can survive extreme conditions. Modeling such living systems is already complex in itself. Add to that the multitude of factors influencing something as intricate as climate, and manipulating - let alone interpreting - the mathematical and computational representations of these systems becomes even more arduous. To be able to anticipate, control and optimize the evolution of these systems, the team relies on the powerful mathematical tools of control theory (see inset). The gain is well worth every pain: mastering this complexity means depolluting without waste. It means recycling resources efficiently in terms of emissions, costs, and renewable energy. It holds the promise of reversing the wastewater cycle into the virtuous circular economy.
Sneak peek into underwater friendships
Ready, set, go! You now find yourself immersed in a pool filled with greenish-brown water. Don’t panic - though it’s full of contaminants, this pool holds great promise. Adjust your mask and see: tiny microalgae and bacteria are gravitating around molecules of phosphorus and nitrogen. Let's zoom in on everybody's role:
- Phytoplankton
They thrive on industrial carbon dioxide emissions, but also love the phosphorus and nitrogen found in wastewater. Allowing phytoplankton to proliferate in these conditions helps recycle these molecules into other valuable compounds: proteins and other molecules essential for producing bioplastics, biofertilizers, and even biofuels.
- Bacteria
They are so fond of the oxygen produced by phytoplankton through photosynthesis that they have every incentive to support the latter. In exchange, bacteria provide the algae with vitamins for their growth and even more carbon dioxide for photosynthesis. What a virtuous cycle!
- Mathematical models and AI
They typically step in when the story gets complicated. With billions of collaboration scenarios possible between billions of species, how do we select and fine-tune the best ones to provide rapid responses to the accelerating climate crisis? Thanks to control theory tools. Thanks to them, the team can determine optimal and feasible operating conditions while ensuring the desired behaviors are sustainable. These tools also help optimize key criteria such as productivity or operational costs. The resulting studies shape a theoretical framework and strategies to make these systems more efficient, breaking ground for startups like Inalve and DareWin.
Analyzing the true environmental impact of industry-grade solutions
Offering new solutions to environmental challenges is great, but being able to assess their true environmental impact is even better. This is usually where surprises arise, hence the team's third and last mission in the realm of Life Cycle Assessment (LCA). This science evaluates the environmental impact of a product or service with the goal of eco-design and reducing its environmental footprint. However, unraveling the canvas that produces a good or service, one thread at a time, requires massive databases. It is up to our research scientists to cross-reference these data to assess, both directly and indirectly, everything a product releases into the environment and its consequences.
“Thanks to this analysis powered by computational modeling, we can assess our own bioprocesses and solutions, but it also allows everyone to evaluate theirs,” explains Olivier. This work has already enabled the team to identify weak links in their artificial microbial ecosystems, such as the pollution resulting from the volume of water that needs to be stirred to stimulate phytoplankton growth. The team thereby came up with an innovative, more sustainable solution made of large spinning rollers covered with biofilm. This mechanism allows the microalgae to grow much faster while requiring less energy. “Instead of stirring water, we decided to turn the wheel” Olivier summarizes. Once the patents were filed, the Inalve startup took over in 2016 to bring the algae to market, namely as a sustainable alternative to fishmeal and soy in aquaculture. Another startup is about to be launched, pushing the scientific horizon even further with the team, with a goal to domesticate natural evolution.
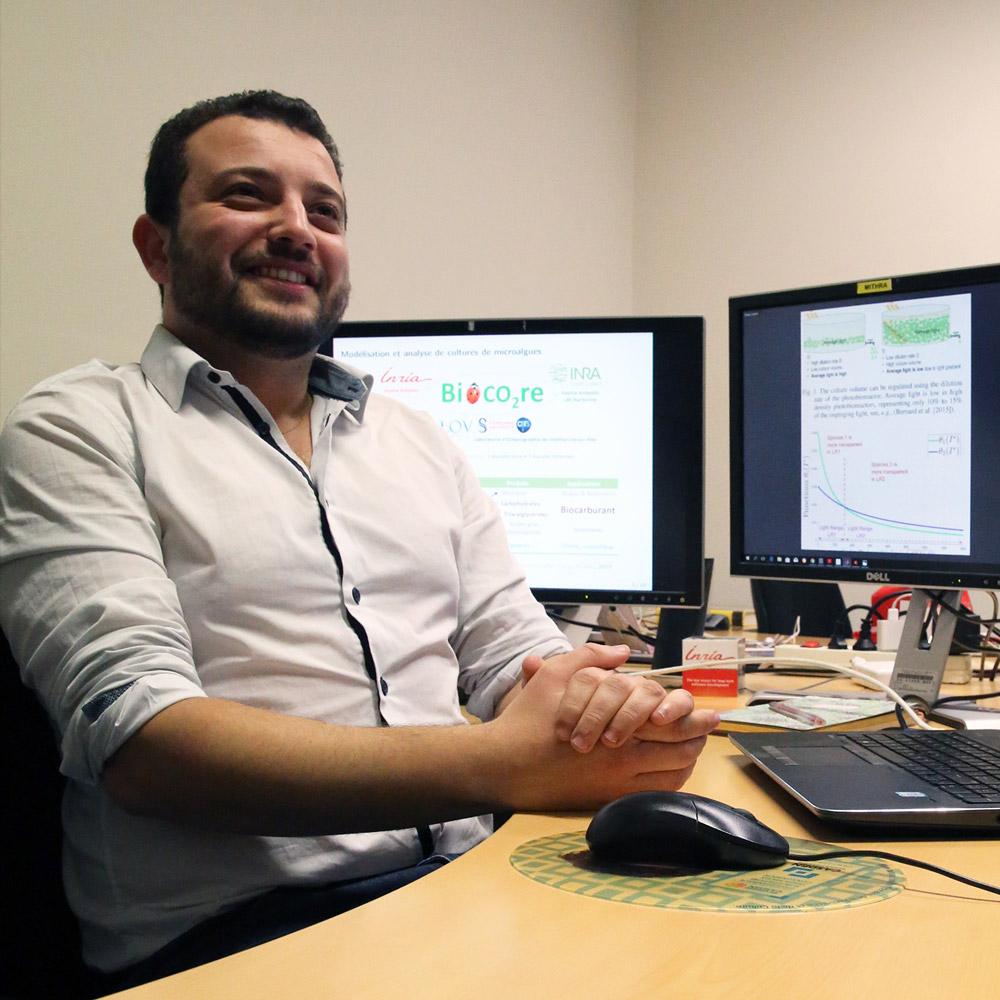
Verbatim
The aptly named DareWin is on a mission to accelerate evolution in a targeted way. Using the natural selection process, it will develop new organisms with optimized characteristics, such as individuals richer in lipids for biofuel production or more efficient in nitrogen recycling. Based on the optimal cultivation conditions established and validated through our models, this approach promotes the rapid growth of the desired organisms. In short, beyond mere ecological predictions, computational modeling allows us to maximize and improve the productivity of the ecosystems we study."
Research Scientisit in Control and Mathematical Biology, Centre Inria d’Université Côte d’Azur
Winner of the i-PhD deeptech startup innovation competition in the Pharmaceuticals & Biotechnologies category, DareWin is literally taking Darwin to the race track. Thanks to its innovative Darwinian dynamic selection approach, the team can increase phytoplankton productivity in a natural way. Proofs of concept show that gains in excess of 100% can be achieved after just a few months of selection.
For instance, if the temperature rises by so many degrees, DareWin will determine the mathematical conditions for selecting micro-organism cells that are more efficient around a given resource. The fact that DareWin's selection strategies don't apply over a century but over a year is taking these calculations to the next level of complexity. But it also means fast, working solutions can be brought to environmental and agricultural emergencies, such as the need to offer sustainable alternatives to GMOs. Again, this is just one example of the multiple horizons that the bioprocesses developed by our research team are destined to open up in the face of increasingly complex environmental challenges.
At the end of the day, aren't mathematicians and biologists like bacteria and phytoplankton? They both have a million reasons to team up. But as we are bidding farewell to Olivier and his conductors of the invisible, we are left with one tiny question unanswered...
'Mathematical monsters, underwater friendships, deep sea learning: what is the most powerful in it all?'
'There is nothing more powerful in my eyes than being able to bridge mathematics to the world’s most pressing needs,' concludes Olivier.
Food for thought...
Seaweed or Salmon? That is the question
Do you know why we all go after fish? For the long-chain omega-3 polyunsaturated fatty acids that fish are...incapable of producing. What they do is simply eat zooplankton that have ingested phytoplankton, which in turn produce all the nutrients our brains need. So wouldn't it be more efficient and sustainable - let alone simpler - to indulge in seaweed at the restaurant? Algae thermidor, por favor!